Coenzyme Q10, Vitamin E and Polyvitamin B: an exploratory double-blind randomized cross-over study in Phelan-McDermid Syndrome
Abstract
Aim: Defective mitochondrial function and increased oxidative stress have been documented in Autism Spectrum Disorder (ASD) and Phelan-McDermid syndrome (PMS), one of the best-known monogenic forms of ASD. The purpose of this exploratory, double-blind, randomized cross-over trial (RCT) is to verify the efficacy and safety of a “metabolic support therapy” (MST) in PMS, while defining the experimental methodology most apt at maximizing sensitivity and reliability.
Methods: A total of 31 PMS patients completed 4 months of Coenzyme Q10 (50/100 mg b.i.d.) + vitamin E (30/60 mg/d) + polyvitamin B ("active compound") vs. 4 months of only Vitamins E and B ("active comparator"). To explore their sensitivity and reliability, four primary outcome measures were used: VABS, CARS, CGI-I, and VAS. Secondary outcome measures span adaptive behaviors, social cognition, autism, problem behaviors, quality of life (QoL), communication, and comorbidities.
Results: CoQ10+vit. E and B yielded significantly greater improvement in several measures of cognition and adaptive functioning, motor skills, and stereotypic behaviors compared to vit. E and B only. Maternal QoL was especially improved in the presence of CoQ10 (P < 0.004). Time x Treatment interactions in CGI-I and VAS "restricted interests" scores support positive contributions also by vitamins E and B. Side effects, including hyperactivity, insomnia, and irritability, were mild, rare, and did not differ between treatment periods.
Conclusion: MST may produce small-to-moderate improvement, especially in motor skills, social motivation, adaptive behaviors, responsiveness to environmental stimulation, and stereotypic behaviors in up to approximately 70% of PMS patients. A targeted confirmatory RCT contrasting Q10+vit E and B vs. inactive placebo is now warranted.
Keywords
INTRODUCTION
Phelan-McDermid syndrome (PMS, OMIM#606232) is a rare genetic neurodevelopmental disorder mainly characterized by global developmental delay, severe deficits in expressive language, and intellectual disability (ID)[1-4]. The prevalence of PMS has been estimated in the range of 2.5-10 cases per million births[5]. However, the prevalence of PMS is likely to be underestimated and over 3,000 individuals self-identified as PMS patients worldwide are registered in the “Phelan-McDermid Syndrome International Registry” of the PMS Foundation (https://www.pmsf.org/international/)[5]. Autism Spectrum Disorder is present in as many as 30%-80% of PMS patients, while at least 0.5%-2.0% of autistic individuals carry a genetic abnormality related to PMS in chr. 22q13[6-8]. A wide range of other neurological and behavioral signs/symptoms may also be present, such as muscle hypotonia and deficits in motor coordination, seizures, structural brain abnormalities, gastrointestinal symptoms, renal malformations, and minor dysmorphic features[1-4,7,8]. Recently, other psychiatric disorders were found to be associated with PMS, including catatonia, mood, and psychotic disorders[9]. Lasting regressions in communication, self-care, and motor function beginning within 3 years of the onset of psychiatric illness were found to exert a very negative impact on clinical prognosis[10].
The pathophysiology of PMS is due to a spectrum of genetic anomalies in the terminal region of the long arm of chromosome 22, ranging from disruptive single-nucleotide variants to ring chromosomes and large deletions affecting multiple genes[2]. SHANK3 (OMIM *606230), located in the distal portion of the long arm of chromosome 22, is the strongest candidate gene to determine PMS[3,11]; however, genotype-phenotype correlations in PMS are complex, as even interstitial deletions sparing SHANK3 have been described in some PMS patients[12]. The size of PMS-causing deletions can be extremely variable, ranging from 0.22 to 9.22 Mb[8], and a multitude of genes distributed along this terminal region may contribute to phenotypic heterogeneity and clinical severity[13]. Furthermore, rare autosomal recessive forms of mitochondrial disorders have been found to emerge from the coincidence of a large PMS-causing deletion on one chr. 22q13 allele and of a missense mutation or small deletion not detectable by array-CGH located on the other allele[14]. The larger chr.22 segment deleted in PMS hosts several genes involved in mitochondrial function (SCO2, CHKB, TYMP, TRMU, NDUFA6, SAMM50, SULT4A1)[13,15]. Abnormalities in electron transport chain (ETC) complex activity have been detected in 30/51 (59%) individuals with PMS[15], supporting the hypothesis that mitochondrial dysfunction could contribute to the pathological process and phenotypic expression of PMS in many patients.
Behavioral and psychological/physical treatments are considered the first line intervention, because there is no pharmacological compound yet proven effective in ameliorating the core signs/symptoms of PMS[16]. Pharmacological treatment strategies adopted to improve psychiatric comorbidities and sleep disorders are not different from those prescribed in the general population[17], but responses to traditional psychiatric intervention are variable and sensitivity to the development of side effects is seemingly high[9].
Coenzyme Q10 (CoQ10, ubiquinone) is a lipid-soluble quinone present in the highest concentrations in brain, heart, kidney, and liver tissue[18]; tissue levels reflect the extent of metabolic activity and energy of each tissue and organ[19]. CoQ10 can be found in three distinct redox states: oxidized (ubiquinone), semiquinone (ubisemiquinone), and reduced (ubiquinol)[18]. It is synthesized by human cells and can also be partly obtained from dietary sources (on average 5 mg/day)[20]. In the latter case, Q10 ubiquinone is reduced to Q10 ubiquinol in the liver following gut absorption. Both ubiquinone and ubiquinol are currently available as dietary supplements[21]. CoQ10 plays an important role in supporting energy production in living cells[19], and orally administered CoQ10, in the presence of partial tissue deficiency, can boost electron transfer and ATP synthesis[22-24]. More specifically, CoQ10 is present in two intracellular pools, a protein-bound pool (30%) and a free-cytosolic pool[21]. The former plays a pivotal role in the electron chain transport system responsible for the synthesis of ATP, whereby CoQ10 transfers electrons from complex I and II to complex III by shuttling in its redox cycle[21]. Through this mechanism, Q10 supplementation can boost energy levels and reduce the risk of mitochondrial damage due to excessive oxidative stress[21-25]. The cytosolic pool participates in other metabolic processes, most importantly playing an additional antioxidant role by protecting lipids and other cell components, while also restoring reduced levels of other antioxidants[21]. Overall, increased energy production and antioxidant capacity are predicted to limit the damage generated by the neuroinflammation and excitotoxicity well documented in ASD brains, ultimately leading to excessive neuritic pruning and/or cell apoptosis[26,27]. Interestingly, serum coenzyme Q10 levels were found to interact with genetic predisposition to a variety of neuronal diseases in a genome-wide association study[28]. Moreover, converging evidence strongly supports its neuroprotective effects[22,29,30] and numerous disease processes can benefit from oral administration of CoQ10, including neurodegenerative diseases[24,31-34].
In an attempt to transfer this knowledge “from bench to bedside”, we designed a “metabolic support therapy” (MST) encompassing Q10 ubiquinol or CoQ10 (50-100 mg b.i.d.) + vit. E (30-60 mg/die) + poly vitamin B (B50 complex, 1/2 or 1 caps/die), under the hypothesis that its metabolic, antioxidant and neurochemical effects could at least partly improve the clinical signs/symptoms of neurodevelopmental disorders. Recently, we performed a retrospective chart review reporting small-to-moderate improvement in cognitive and adaptive functioning, social interaction and motor coordination in 45/59 (76.3%) patients with different neurodevelopmental disorders, with excellent tolerability[35]. These results were especially promising in five PMS patients, all displaying some measurable degree of clinical response[35].
Based on the evidence summarized above, we have now designed and performed an exploratory 32-week randomized, double-blind, placebo-controlled, cross-over trial (RCT), with (A) the primary aim to evaluate the efficacy and safety of MST in PMS patients and to begin dissecting the selective contribution of CoQ10 to efficacy and tolerability, as compared to vitamins alone; (B) the secondary aim to identify a panel of psychodiagnostic tools endowed with sufficient sensitivity to reliably detect a small-to-moderate effect size in a severely-compromised patient population. This information will then be used to design a confirmatory RCT applying a targeted strategy.
METHODS
Study design
This double-blind, randomized cross-over, placebo-controlled study assessed the efficacy and safety of an MST with CoQ10, vitamin E and complex-B vitamins in PMS. All procedures contributing to this work comply with the ethical standards of the relevant national and institutional committees on human experimentation and with the Helsinki Declaration. The Ethical Committee of the University of Messina (Messina, Italy) approved the study (prot. n. 15/18, approved on May 2nd, 2018). The parents of all patients enrolled in this study were informed about potential adverse reactions. Written informed consent was obtained from all caregivers for their child's participation. This clinical trial adheres to CONSORT guidelines and has been registered at https://clinicaltrials.gov/ with NCT n. 04312152.
The experimental design of this trial is displayed in Figure 1. Briefly, the total duration of the trial was 8 months. Each patient received an active compound [CoQ10 + Vit. E + polyvit. B] for 4 months and active comparator [Vit. E + polyvit. B] for 4 months. Half of the sample received the active compound during period I (months 1-4) and the active comparator during period II (months 5-8), while the remaining half assumed the active comparator during period I and active compound during period II [Figure 1]. More specifically, following a medical screening visit, enrolled patients were double-blindly randomized according to a permuted two-block design, to receive either active compound or active comparator (Period I) and then were switched to the other treatment condition (Period II); each treatment period lasted for 4 months. Initially eligible subjects underwent a complete clinical and psychometric assessment (T0), which was repeated at the end of each 4-month treatment period [T1 and T2 in Figure 1]. Three investigators (L.T., G.C., T.DB.), never involved in clinical assessments nor in direct contact with patients and caregivers, were in charge of treatment allocation. To monitor compliance, unused capsules were retrieved and counted at each time point. One investigator (A.R.), not involved in clinical assessments, interacted with caregivers as needed throughout the study, for any medical need and for issues regarding the trial itself. Families were requested to refrain from commenting on their experience and purported treatment efficacy on social media.
Participants
Inclusion and Exclusion Criteria
Participants were required to hold a diagnosis of PMS due to a genetically documented deletion of human chromosome 22q13.3 or a disruptive mutation in the SHANK3 gene. Other inclusion criteria were: (1) age between 2 and 40 years old; (2) Children's Global Assessment Scale (CGAS) score between 45 and 59 at baseline; (3) medication regimen stable for at least three months prior to enrolment and kept constant throughout the 8-month duration of the trial; (4) behavioral intervention started at least 3 months prior to enrolment and kept unchanged throughout the 8-month duration of the trial; (5) written informed consent provided by both parents or a legally authorized patient representative; (6) parents and guardian able to understand and comply with the experimental protocol.
Potential participants were excluded in the presence of any of the following: (1) known genetic syndromes other than PMS (for example, Rett syndrome, fragile-X syndrome, etc.); (2) serious medical illnesses (chronic renal disease, severe liver disease, cardiovascular disorders, uncontrolled hypertension with systolic pressure values > 170 mm Hg and diastolic pressure > 100 mm Hg, malignant tumors, HIV infection); (3) history of acute cerebrovascular disease; (4) history of stomach bleeding or active peptic ulcer; (5) documented allergies, hypersensitivity or intolerance to one of the excipients of the experimental compound or comparative product; (6) treatment with anticoagulants. Seizures were not a cause of exclusion and were categorized as “frequent” if occurring more often than once every 6 months, “rare” if occurring at a lower frequency, or otherwise “absent”.
Trial interruption criteria include (1) the onset of a severe medical condition during the trial; (2) changes in psychopharmacological or behavioral treatment lasting longer than two weeks during the 8-month duration of the trial; (3) medical conditions requiring anticoagulant treatment started during the trial.
Patient sample
Sixty-six PMS patients were screened for eligibility at the Interdepartmental Program “Autism 0-90” of the “G. Martino” University Hospital in Messina, Italy, between March 2019 and September 2021. The CONSORT flow-chart of the study is depicted in Figure 2. Five patients did not meet inclusion criteria, five already received open treatment with CoQ10/ubiquinol and vitamins[34], and twenty-one refused participation. The remaining 35 PMS patients were recruited. Comorbid neurodevelopmental disorders were diagnosed based on DSM-5 criteria[36]. The total sample was stratified by sex and randomly assigned to receive the active compound [CoQ10+VitE+VitB] either during the first or the second administration period. Two patients, one per group, dropped out due to non-compliance; hence a total of 33 PMS patients completed the entire trial and were included in the statistical analysis [Figure 2].
Figure 2. CONSORT flow-chart. Sixty-six PMS patients were assessed for eligibility. Thirty-five of them were recruited and randomized into groups receiving either active compound (n = 18) or active comparator (n = 17) during the first 4 months. Thirty-three patients completed the entire study, whereas one patient dropped out of each treatment group during the first 4-month period.
Intervention
The active compound encompasses CoQ10 (50-100 mg b.i.d.), vitamin E (30-60 mg b.i.d.), and B-group vitamins, including nicotinamide, dexpanthenol, riboflavin-5’- sodium phosphate, inositol, pyridoxine hydrochloride, and cyanocobalamin, while active comparator is devoid of CoQ10 and includes vitamins E and B only [Table 1]. Active compound and active comparator were overencapsulated to preserve blinding and administered orally twice daily (BID) during the trial. If patients were unable to swallow capsules, parents were allowed to open the capsules and immediately administer their contents in a small quantity of juice or soft drink. Two different doses were prescribed based on body weight below or above 20 kg [Table 1]. Vitamins and minerals were dosed considering the maximum daily intake allowed in food supplements by the Italian Ministry of Health (Italian Ministry of Health, Directorate-General for Hygiene and Food Safety and Nutrition, 2021) and on the basis of our prior retrospective analysis[35].
Composition of the active compound. The active comparator is devoid of CoQ10 and contains all the remaining components
Compounds | Daily dose by patient weight | |
≤ 20 kg | > 20 kg | |
CoQ10 | 50 mg | 100 mg |
Vitamin E | 30 mg | 60 mg |
Vitamin B1(thiamine) | 1.05 mg | 2.10 mg |
Vitamin B2 (riboflavin HCI) | 1.2 mg | 2.4 mg |
Vitamin B3 (Niacin) | 9 mg | 18 mg |
Vitamin B5 (Pantothenic acid) | 4.5 mg | 9 mg |
Vitamin B6 (Pyridoxine) | 1.5 mg | 3 mg |
Vitamin B12 (Cyanocobalamine) | 4.5 μg | 9 μg |
Folic acid | 0.1 mg | 0.2 mg |
Biotin | 0.1125 mg | 0.225 mg |
CoQ10 | 50 mg | 100 mg |
Patient assessment and outcome measures
At baseline, each patient underwent a comprehensive medical evaluation, including physical and neurological examination, routine hematology and blood chemistry. We collected family, developmental, educational, medical and psychiatric history. A detailed mental status examination was also performed, applying DSM-5 diagnostic criteria[36]. The complete battery of neuropsychological and behavioral assessments performed at T0, T1, and T2 is summarized in Table 2.
Clinical and psychodiagnostic assessment performed at each time point
Clinical Assessment | Psychodiagnostic assessment | |
T0 Baseline | √ Physical and neurological examination √ Family, developmental, educational, medical and psychiatric history √ Auxological parameters (height, weight, head circumference) √ Routine hematology and blood chemistry |
√ Questionnaires: SRS, RBS-R, ABC, CPRS, CBCL, SSP, QOLA, WHOQOL, SCQ. √ Clinical scales: VAS, CGAS and CGI-S (clinician ratings); VAS (parental ratings) √ Test: ADOS2; ADI-R; GMDS-III or cognitive test; VABS-II; CARS2. |
T1 | √ Physical and neurological examination √ Anamnestic data (Period I) √ Measures of therapy adherence √ Drug safety monitoring |
√ Questionnaires: SRS, RBS-R, ABC, CPRS, CBCL, SSP, QOLA, WHOQOL, SCQ. √ Clinical scales: VAS, CGAS, and CGI-I (clinician ratings); VAS and CGI-I (parental ratings) √ Test: VABS-II; CARS2. |
T2 | √ Physical and neurological examination √ Anamnestic data (Period II) √ Measures of therapy adherence √ Drug safety monitoring |
√ Questionnaires: SRS, RBS-R, ABC, CPRS, CBCL, SSP, QOLA, WHOQOL, SCQ. √ Clinical scales: VAS, CGAS, and CGI-I (clinician ratings); VAS and CGI-I (parental ratings) √ Test: VABS-II; CARS2. |
Intellectual/developmental level was assessed using the Griffiths Developmental Rating Scales III, Wechsler Intelligence Scale for Children - Fourth Edition (WISC-IV), or the Leiter III scale, depending on age and expressive language development. Independent evaluators (B.F., B.M., T.G.), blind to treatment conditions and safety data, performed neuropsychological and behavioral testing. Given the exploratory nature of this RCT and the lack of preliminary data on sensitivity to change-over-time of psychodiagnostic scales in PMS patients, multiple primary and secondary measures were applied, selected among those previously used in individuals with PMS, ASD, intellectual disability, or displaying greater sensitivity in RCTs of fragile X syndrome[1,4,37-39].
Primary outcome measures include:
A. Vineland Adaptive Behaviour Scales-II (VABS-II)[40], a standardized semi-structured parental interview to measure adaptive functioning in real life. Standard scores have a mean of 100 and a standard deviation of 15.
B. Childhood Autism Rating Scale 2 (CARS2)[41], for trained clinicians to rate the presence and severity of signs and symptoms of ASD by direct observation of the child. Scores range from 15 to 60 (< 30, non-autistic; 30-36.5, mild to moderate autism; 37-60, severe autism).
C. Clinical Global Impression - Severity/Improvement scale (CGI-S/I)[42], a 7-point scale for the clinician to quantify illness severity (CGI-S) at baseline, patient improvement/worsening (CGI-I) at follow-up, and treatment side effects.
D. Visual Analog Scales (VAS)[43,44], spanning 16 items listed in Table 3, and ranging from 0 = “no impact” to 10 = “severe impact”. Items include all core ASD symptoms based on DSM-5 criteria, fine and gross motor function, as well as an additional set of behaviors reflecting different aspects of social cognition (imitation, play, joint attention, enjoyment in shared play). The latter variables were chosen for their relevance in neurodevelopmental disorders and based on our prior observations in patients receiving an open treatment with MST[35]. VAS was completed both by clinicians and parents at each time point.
Visual Analog Scales (VAS) used to assess core autism symptoms based on DSM-5 criteria for Autism Spectrum Disorder[36], and additional behaviors of clinical relevance. Each item ranges from 0 (symptom absent) to 10 (extreme severity)
ITEMS | DEFINITION |
Socio-emotional skills | Deficits in socio-emotional reciprocity, ranging, for example, from abnormal social approach and failure of normal back-and-forth conversation, to reduced sharing of interests, emotions, or affect, to failure to initiate or respond to social interactions |
Non-verbal communication behaviors | Deficits in nonverbal communication behaviors used for social interaction, ranging from poorly integrated verbal and nonverbal communication to abnormalities in eye contact and body language or deficits in understanding and use of gestures |
Verbal communication behaviors - expressive language | Deficits in expressive language and verbal communication behaviors used for social interaction |
Verbal communicative behaviors - receptive language | Deficits in receptive language and verbal communication behaviors used for social interaction |
Theory of mind | Deficits in developing, maintaining, and understanding relationships, ranging, for example, from difficulties adjusting behavior to suit various social contexts, to difficulties in sharing imaginative play or in making friends, to absence of interest in peers |
Stereotyped or repetitive motor movements | Stereotyped or repetitive motor movements, use of objects, or speech |
Sameness | Insistence on sameness, inflexible adherence to routines, or ritualized patterns of verbal or nonverbal behavior |
Restricted interests | Highly restricted, fixated interests that are abnormal in intensity and/or focus |
Hold an object in hand | Strong attachment or concern for objects which are often held in the hand for a long time or continuously |
Sensory issues | Hyper- or hypo-reactivity to sensory input or unusual interest in sensory aspects of the environment |
Imitation | Deficit of imitation with objects and/or imitation of actions and/or verbal imitation. |
Play | Quality of spontaneous play (i.e., functional/imitation play, symbolic/representational play) |
Fine-motor skills | Motor control deficits in small movements of the hands and fingers, as well as in small muscles of the face and mouth (tongue) |
Gross-motor skills | Motor control deficits in global movements, such as pushing and manipulating large objects, rolling around, climbing, and jumping. |
Joint attention | Lack of ability to coordinate attention with another person, i.e., you point at an object and see if the child looks in that direction and then turns his/her gaze back towards you. |
Enjoyment in shared play | Lack of participation in play activities with others, employing exchanges of glances and smiles. |
Secondary Outcome Measures include:
A. Children's Global Assessment Scale (CGAS)[45], which provides a global measure of the level of functioning in children and adolescents. The measure provides a single rating on a 0-100 scale, with higher scores indicating better functioning.
B. Social Responsiveness Scale (SRS)[46], a 65-item questionnaire used to assess social impairment, communication deficits, and repetitive behaviors in children and adolescents 4-18 years old. Autism is severe, moderate, or mild when T-scores are > 75, 66-75, or 60-65, respectively. Scores < 60 are not clinically significant.
C. Repetitive Behaviors Scale - Revised (RBS-R)[47], a 44-item parental questionnaire used to assess repetitive behaviors in children 6-17 years old. Each behavior is rated on a 0-3 point scale to measure increasing symptom severity, as does the overall global score ranging from 0-100.
D. Aberrant Behaviour Checklist (ABC)[48], a 58-item parental questionnaire used to assess problem behaviors rated on a 0-3 point scale to measure increasing severity.
E. Short Sensory Profile (SSP)[49], a 38-item parental questionnaire assessing sensory processing, sensory modulation, and behavioral/emotional responses. The overall score ranges from 0 to 190, with lower scores reflecting greater symptom severity.
F. Conners' Parent Rating Scale-Revised (CPRS-R)[50], a 48-item parental rating scale used to evaluate the presence and intensity of hyperactivity/inattention, impulsivity, and externalizing behaviors. Each item is rated on a 0-3 point scale to reflect increasing symptom severity.
G. Child Behaviour Checklist (CBCL)[51,52], which provides parental ratings for 20 and 120 items regarding competence and behavioral problems, respectively, in youth aged 6-18 years old. Each item is scored 0-2 to reflect symptom severity or frequency. Standard scores are scaled so that 50 is average for the youth's age and gender, with a standard deviation of 10 points.
H. The Quality of Life in Autism Questionnaire (QOL-A)[53], which measures the parental quality of life either broadly (part A, 28 items, score range 28-140) or specifically related to the ASD affecting their offspring (part B, 20 items, score range 20-100). The total score range is 48-240, with higher scores indicating better quality of life.
I. The World Health Organization's Quality of Life Questionnaire (WHOQOL)[54], which is used to assess parental quality of life in four domains: physical, psychological, social, and environmental. The score can range from 15 to 105, with a higher score indicating better quality of life.
Safety and Adverse Events
Adverse Events (AEs) were monitored during the study by extensive clinical evaluation at each time point, including physical and neurological examination, routine hematology and blood chemistry. Moreover, an investigator (A.R.) blind to treatment and not involved in patient assessments was available to interact with families by phone throughout the study for prompt detection and management of potential adverse effects in-between assessments. The AEs were documented for severity, duration, and management.
Statistical analyses
The statistical analysis was carried out using repeated measures mixed models. The dependent variables were the post-treatment outcome values, whereas the independent variables were treatment (active compound vs. active comparator), time (Period II vs. Period I), and sequence (the active compound in the first period vs. active compound in the second period). A random intercept term was used in the model to account for repeated measurements over the same individuals. Results for numerical variables were reported as the mean difference (MD) from a linear mixed model, whereas for binary or ordinal categorical variables as the odds ratio (OR) from a logistic mixed model or as the cumulative odds ratio (cOR) from an ordinal logistic mixed model, respectively. To describe the effect of treatment in Period I and Period II, standard linear, logistic, and ordinal logistic models were used, with post-treatment values as the dependent variable and treatment as the independent variable. All linear models were also adjusted for pre-treatment outcome values. Uncertainty in estimates was described using 95% confidence intervals (CI). Given the exploratory nature of this study, nominal P-values are presented and statistical significance is set at nominal P < 0.05 without controlling for multiple comparisons. Effect modification related to age (considering two subgroups: < 9 and ≥ 9 years) was assessed by significance testing of the treatment x age interaction term in a separate model for each primary outcome variable reaching nominal significance. Analyses were performed using the R 3.6.3 statistical software[55].
RESULTS
The study was completed by 33 patients whose demographic, genetic, and clinical characteristics are summarized in Table 4. The sample was randomized to receive the active compound either during the first
Demographic and clinical characteristics of the sample (n = 33)
Characteristics | n | Mean ± S.E.M. (range) or % |
Age in yrs | 33 | 11.87 ± 0.33 (3-37) |
● 0-9 yrs | 19 | 57.6% |
● 10-18 yrs | 9 | 27.3% |
● ≥19 yrs | 5 | 15.2% |
Sex | ||
● M | 17 | 51.5% |
● F | 16 | 48.5% |
● M : F ratio | 1.06 : 1 | |
Genetics features: | ||
● chr 22q13.3 deletion | 26 | 78.8% |
● SHANK3 mutation | 4 | 12.1% |
● Ring chromosome | 3 | 9.1% |
● Mosaicism | 2 | 6.1% |
DSM-5 Diagnosis: | 32 | 97.0% |
● Global Developmental Delay (GDD) or Intellectual Disability (ID) | 19 | 57.6% |
● GDD or ID + Autism Spectrum Disorder | 13 | 39.4% |
IQ†: | ||
● > 70 | 3 | 9.1% |
● | 30 | 90.9% |
CGI-Severity score‡: | ||
● Borderline ill | 2 | 6.1% |
● Mildly ill | 1 | 3.0% |
● Moderately ill | 11 | 33.3% |
● Markedly ill | 14 | 42.4% |
● Severely ill | 5 | 15.2% |
Behavioral regression history: | ||
● Present | 10 | 30.3% |
● Absent | 23 | 69.7% |
Bipolar Disorder: | ||
● Present | 7 | 21.2% |
● Absent | 26 | 78.8% |
Epilepsy: | ||
● Frequent | 1 | 3.0% |
● Rare | 5 | 15.2% |
● Absent | 27 | 81.8% |
Developmental Coordination Disorder: | ||
●Gross-motor skills | 33 | 100.0% |
●Fine-motor skills | 30 | 90.9% |
Expressive language (single words): | ||
● Normal development (<18 mo) | 2 | 6.1% |
● Developmental delay (> 18 mo) | 11 | 33.3% |
● Lost after normal development | 1 | 3.0% |
● Lost after developmental delay | 3 | 9.1% |
● Never acquired | 16 | 48.5% |
Mean VABS scores (Adaptive skills)§: | ||
● Communication | 33 | 41.8 ± 3.1 (19-72) |
● Daily living skills | 33 | 49.0 ± 3.1 (21-81) |
● Socialization | 33 | 52.4 ± 2.8 (20-79) |
● Motor skills | 10 | 58.2 ± 3.1 (37-72) |
● Composite score | 33 | 47.0 ± 2.7 (19-72) |
Ongoing psychopharmacological treatment: | ||
● Antiseizure medications ● Atypical Antipsychotics | 5 3 | 15.2% 9.1% |
● Lithium carbonate | 1 | 3.0% |
● Melatonin | 3 | 9.1% |
● Dietary supplements | 1 | 3.0% |
Regarding primary outcome measures, several measures reached nominal significance in favor of the active compound [CoQ10 + Vit. E + polyvitamin B] producing greater improvement than the active comparator [Vit. E + polyvitamin B] [Table 5A for summary statistics and Figure 3, depicting single patients]. Specifically, greater positive responses were recorded mainly in:
Figure 3. Single-patient line graphs for primary outcome measures reaching significantly greater improvement with active compound (thick lines) vs. active comparator (thin lines) [see Table 5A]: (A) VABS Motor Skills scores; (B) VAS Enjoyment in shared play; (C) VAS Stereotyped behaviors (parent-rated); (D) VAS Stereotyped behaviors (clinician-rated). The patient legend of panel B also refers to panels C and D. Higher scores in panel A indicate better motor skills; in panels B-D, higher scores indicate greater symptom severity.
Primary outcome measures reaching nominal significance, indicating (A) greater improvement with active compound [CoQ10 + Vit. E + polyvit. B] vs. active comparator [Vit. E + polyvit. B], or (B) Time × Treatment interactions with active compound displaying greater efficacy when administered during period II, but not during period I (also see Figure 4, A and B).
Subscale | MD or cOR | 95% CI | Nominal P-value | ||||
A | VABS Motor Skills VAS Stereotyped or repetitive motor movements (rated by parents) VAS Stereotyped or repetitive motor movements (rated by clinicians) VAS Enjoyment in shared play (rated by parents) | +6.81 -0.79 -0.97 -0.54 | +1.89 -1.45 -2.06 -0.99 | +11.72 -0.13 0.12 -0.09 | 0.0147 0.0198 0.0770 0.0199 | ||
B | VAS Restricted Interest (rated by parents) | - T1 | +0.42 | -0.18 | +1.01 | 0.1701 | |
- T2 | -1.40 | -2,76 | -0.04 | 0.0434 | |||
CGI-Improvement | - T1 | +0.78 | +0.21 | +2.94 | 0.7150 | ||
- T2 | +4.55 | +1.12 | +20.00 | 0.0336 |
(a) Motor skill deficits, with VABS motor skill displaying a sizable improvement (MD +6.81, 95%CI: +1.89; +11.72, P = 0.0147). This statistic includes only 10/33 patients whose age falls within the range for VABS motor skill (0-6 years), but it is paralleled by non-significant improvements in VAS gross- and fine-motor skills collected for all 33 patients, reaching a P = 0.058 in clinician-scored VAS for fine-motor skills. These results are also consistent with clinical observation of widespread small-to-moderate improvement, especially in fine-motor function. This positive effect was surprisingly most evident in three highly compromised patients initially wheelchair-bound due to very large deletions spanning from SHANK3 to TCF20 and beyond: all three became able to hold up and use fork and spoon during meals; for one, it became feasible to sit up and undertake sessions of mild horse-assisted intervention.
(b) "Enjoyment in shared play", i.e., participation in play activities with others, employing exchanges of glances and smiles (VAS, P = 0.0199). This variable exemplifies one of the benefits most consistently reported by parents, namely increased responsiveness to environmental stimulation and greater social motivation;
(c) Repetitive behaviors were significantly reduced according to parents (VAS, P = 0.0198), with a similar trend also observed by clinicians (VAS, P = 0.0770). This observation describes a consistent subgroup of patients and not a small subset because at T0, parents reported the presence of motor stereotypies in 27 (81.8%) patients, and during the intake visit, motor stereotypic behaviors were directly observed by the clinician in 15 (45.5%) patients.
No effect modification by age was detected for enjoyment in shared play (P = 0.2896) and repetitive behaviors (med P = 0.5297, par P = 0.5656), whereas the number of observations for VABS motor skills was not sufficient to allow reliable testing of age effects.
CGI-I scores were consistent with both active compound and active comparator producing overall improvement in a sizable number of cases, with a slight, non-significant advantage for active compound. However, CGI-I scores and parent-rated VAS “Restricted Interests” scores yielded significant Time × Treatment interactions [Table 5B, Figure 4]. In fact, both variables displayed no difference when the active compound was administered during the first four months (Period I), whereas significantly greater efficacy was observed when active compound was administered during Period II, following four months of administration of vitamins E and B complex [Table 5B, Figure 4]. Finally, secondary outcome measures revealed a very significant positive influence of active compound on parental quality of life, with over four points of mean increase in maternal WHOQOL scores (MD +4.72, 95%CI: +1.34; +8.11, P = 0.0079), and paternal scores displaying a similar trend (MD +3.61, 95%CI: -0.40; +7.63, P = 0.0755). None of the single WHOQOL items reached statistical significance. Subjective parental reports describe their child as becoming more alert, more responsive to parental requests possibly due to improved receptive language skills, displaying a better mood and greater social motivation, and overall better adapted to family life and in school.
Figure 4. Time x Treatment interaction for changes in (A) CGI-Improvement rated by clinicians; and (B) VAS “Restricted interests” rated by parents. For both variables, active compound produces significantly greater improvement when administered during Period II, but not during Period I. CGI-I scores: 1 = Very much improved, 2 = Much improved, 3 = Minimally improved, 4 = No change, 5 = Minimally worse, 6 = Very much worse.*P< 0.05.
Side effects were rare, minor, transient and did not significantly differ between active compound and active comparator [Table 6]. The most frequent were hyperactivity and sleep disturbances, irritability, and increased appetite. Among six patients with a history of seizures [Table 4], none displayed a clinically meaningful change in seizure frequency and/or severity during either treatment period compared to baseline. No serious adverse event occurred and no participant had to stop treatment because of intolerable side effects.
Number of patients reporting side effects
Hyperactivity + sleep disturbance | Hyperactivity + increased appetite | Increased appetite and/or weight gain | Irritability | TOTAL | |
CoQ10 +
Vit. E + polyvit. B | 2 | 0 | 0 | 1 | 3 |
Vit. E + polyvit. B | 1 | 1 | 1 | 1 | 4 |
DISCUSSION
This is the first clinical trial on the effects of an MST, encompassing CoQ10, Vitamin E, and Polyvitamin B, in Phelan-McDermid Syndrome (PMS). In addition to being a relatively frequent cause of intellectual disability, PMS represents one of the most important syndromic forms of ASD. In fact, our sample includes 13 (39.4%) patients who also satisfy DSM-5 diagnostic criteria for ASD, in agreement with the 30%-80% range reported in the Literature[6-8]. The present study represents a timely follow-up of our recent retrospective chart review, documenting the potential efficacy of MST in a variety of neurodevelopmental disorders, and includes five PMS patients who, surprisingly, all display some degree of clinical improvement[35]. Despite the exploratory nature of this RCT, the comparison between full MST vs. "vitamins only" provides further evidence pointing towards the beneficial effects of CoQ10 as part of MST in PMS. Clinical improvement in our PMS cohort appears to mostly occur in the domains of motor skills, cognition, responsiveness to environmental stimuli (adaptive functioning), and stereotypic behaviors [Table 5]. Given the severity and pathophysiology of this genetic syndrome and of neurodevelopmental disorders in general, it is not surprising that most responders to MST appear "minimally improved", with only some patients occasionally appearing "much improved". We did not detect significant effects on language and communication skills, in contrast to our retrospective study[35]. This discrepancy may reflect the more severe language impairment present in PMS compared to other neurodevelopmental disorders, with its documented structural underpinnings[11,56]. Importantly, the positive influence recorded here on parental quality of life with MST compared to vitamins only, especially for mothers, provides converging support to MST efficacy from a different point of observation. Finally, the extreme safety of this therapy is documented by the rare, mild, and easily manageable side effects reported by parents, equally distributed between the active compound and active comparator [Table 6].
Mitochondrial function plays a pivotal role in brain development and central nervous system (CNS) function. Most brain energy supply is generated by mitochondrial oxidative phosphorylation, and each different neuronal cell type presents local mitochondrial adaptation processes reflecting functional heterogeneity[57]. In addition to energy production, mitochondria are involved in neurogenesis and apoptosis, neuronal migration and differentiation, and neuroplasticity by regulating redox homeostasis, protein and lipid mediators, and intracellular calcium levels[58]. Therefore, it is not surprising that mitochondrial dysfunction and oxidative stress appear to be involved in the pathogenesis of many neurodevelopmental disorders and that antioxidants produce clinical improvement in many patients[59-62]. More specifically, Autism Spectrum Disorder (ASD) is frequently diagnosed in PMS patients and often represents their first clinical diagnosis, formulated long before genetic testing[6-8]. ASD represents a wide collection of multiple genetic and/or epigenetic disorders (the "autisms"), sharing socio-communication deficits, repetitive behaviors, insistence on sameness and abnormal sensory processing as their clinical expression end-point[63]. Enhanced oxidative stress and mitochondrial dysfunction represent one of the most replicated abnormalities detected both systemically and in the Central Nervous System (CNS) of autistic individuals[64]. Targeted and untargeted studies of post-mortem brains, plasma, CSF, urines, and peripheral blood mononuclear cells (PBMCs) consistently documented significant oxidative damage to proteins, lipids and nucleotides, reductions in GSH/GSSG ratio, reduced activity levels of redox protection enzymes, including superoxide dismutase 2 (SOD2), glutathione peroxidase (GPx), glutathione-S-transferase (GST), and glutamate-cysteine ligase, reduced activity of respiratory chain complex I and complex IV due to mitochondrial oxidative damage[65-73]. Abnormalities in these redox parameters have been found to be significantly correlated with the severity of autistic behaviors[74,75] and gastrointestinal symptoms[76,77], which are frequent among autistic children[78]. Not surprisingly, the activity of complex I and IV is significantly reduced in as many as 59% of PMS patients, whose clinical features often overlap with those of mitochondrial diseases[79]. In addition to non-specific impairment of energy metabolism, PMS-causing chr. 22q11.3 deletions often span genes playing relevant roles in mitochondrial function[13]. Although oxidative stress response may represent an evolutionarily preserved strategy aimed at boosting resilience[80] and usually represents the consequence and not the primary cause of ASD-associated genetic syndromes[65], reduced ATP production and excessive oxidative damage can potentially exacerbate the dysfunction caused directly by ASD-causing genetic or epigenetic defects, thereby imposing an additional burden. Importantly, redox abnormalities have also been detected in young autistic children and are not correlated with age[69]. Therefore, enhanced oxidative stress and mitochondrial dysfunction represent a "trait-dependent" characteristic present in a consistent number of PMS patients and autistic individuals, regardless of their age and their specific underlying pathogenetic underpinnings. Since it is not yet possible to directly correct the genetic alteration underlying PMS or its major downstream consequences, sustaining mitochondrial function while controlling redox imbalance may represent a viable indirect therapeutic approach, potentially able to ameliorate cognition and adaptive functioning, motor skills and stereotypic behaviors in many patients, albeit with small-to-moderate effects and with large interindividual differences.
This is the first clinical trial to assess the efficacy and safety of CoQ10, vit. E and polyvit. B in PMS. In reference to ASD, three RCTs have evaluated the effects of over 30 supplements including CoQ10, multiple vitamins, minerals such as lithium, N-acetylcysteine, choline, acetyl-L-carnitine and inositol in 20, 55, and 67 autistic children and adults, respectively[81-83]. Several blood biomarkers were measured, including nutritional (vitamins, minerals, and aminoacids) and metabolic parameters (oxidative stress, methylation, sulfation, glutathione, and neurotransmitters). At baseline, the ASD sample showed significantly higher levels of oxidative stress compared to typically developing controls. After 3 months of treatment, these parameters were either improved or even normalized. Additionally, a behavioral improvement was recorded for several symptoms, as well as in mean Parental Global Impressions-Revised scores, especially for the hyperactivity, oppositional and receptive language subscales[82], and nonverbal intellectual ability[83]. These domains largely overlap with the areas of greatest improvement reported in our retrospective chart review of neurodevelopmental patients[35]. However, the administration of over thirty active compounds hampers the possibility of detecting with reasonable certainty specific contributions by CoQ10 to the observed improvement in biochemical and behavioral parameters. In contrast, more focused RCTs demonstrated a sizable reduction in autistic symptoms following 30 wks of high-dose Vitamin C (110 mg/kg)[84] or after high-dose Vitamin B6 (500-1,000 mg)[85]. To our knowledge, only one RCT has selectively supplemented 78 ASD children with placebo, 30 or 60 mg/day of CoQ10 (n = 26 per sample), recording with the higher CoQ10 dose an improvement in several peripheral redox parameters, paired with a mild reduction ASD severity, sleep disorders and gastrointestinal symptoms[86]. This RCT differs from the present study in experimental design (case-control vs. cross-over), duration (3 mo vs. 4 mo of active compound/comparator), CoQ10 dosage (not based on body weight or age in ref. 86), and in the composition of active compound and comparator (inactive placebo in ref. 86, vitamins E and B here). Nonetheless, its clinical outcome appears convergent with our past[35] and present observations.
From a methodological standpoint, multiple features of this RCT define its exploratory nature. On the one hand, they may represent the limitations of the present study, but on the other hand, they will enable investigators to precisely design targeted confirmatory trials in neurodevelopmental disorders. These points can be summarized as follows:
(A) Initially, this study was undertaken assuming that vitamins would have been entirely inactive on the severe clinical picture of PMS. However, this turned out not to be the case. On the one hand, the Time x Treatment interactions observed on CGI-I and VAS “Restricted Interests” scores demonstrate that in many patients, a four-month course of vitamin E and B seemingly paves the path to a greater response to CoQ10 [Figure 4]. On the other hand, a slight but detectable amelioration was recorded after the administration of vitamin E and polyvitamin B to most patients [Figure 4A]. In some cases, the convergent observation by parents, clinicians, and therapists blind to the treatment of a "much improved" therapeutic effect by vitamins E and B is unlikely to represent a placebo effect in a severe syndrome such as PMS. For the above-mentioned reasons, contrary to its initial expectation, this study has become an "active comparator-controlled", randomized, double-blind, cross-over trial, as it would be inaccurate to define it as "placebo-controlled"[87].
(B) It is not customary to apply as many as four different primary outcome measures in an RCT. This strategy was chosen to verify which outcome measures would display the greatest sensitivity in detecting clinical change over time. The low sensitivity of psychodiagnostic scales and questionnaires in detecting clinically meaningful changes not large enough to bring patients outside the ASD spectrum has been one of the major hurdles encountered in experimental pharmacology of genetic syndromes such as Fragile X[39]. Based on our results, clinician-rated CGI-I and parent-rated VAS appear to be the most sensitive measures, followed by the VABS. For example, the outcome of RBS-R and VAS for stereotypic behaviors appears inconsistent. However, mean pre-/post-treatment changes in VAS for stereotypic behaviors were -0.79 and
(C) Placebo effects in behavioral pharmacology can be large enough to obscure the therapeutic effects exerted by the active compound. This is another critical issue, considering that the therapeutic effect size of MST can be realistically expected in the small-to-moderate range[35]. Several strategies potentially able to minimize the size of placebo effects were applied. Clinicians involved in medical visits and psychodiagnostic testing were screened from interactions with parents outside of T0, T1, and T2. Throughout the study, families were free to contact a dedicated investigator not involved in experimental measures whenever necessary. Using this strategy, the number of contacts between families and experimenters was nil or limited to extremely rare and pre-filtered major issues. Participating families were also generally compliant with our request not to comment on the study on social media. Overall, these strategies appeared to yield satisfactory results, although the lack of an inactive placebo here does not allow us to reliably quantify the placebo effect size and estimate their efficacy.
(D) The duration of RCTs is often dictated more by the available budget and other practical considerations than by preliminary efficacy and safety data. The Time x Treatment interactions depicted in Figure 4 suggest that, while 4 months represent a time interval of sufficient duration to produce therapeutic effects, a slightly longer trial duration, if possible, may yield an even greater therapeutic effect size and/or improvements in more areas.
(E) Cross-over studies typically include a one-week wash-out period between the "active compound" and the "active comparator" periods [Figure 1]. This strategy was not applied here under the hypothesis that neurodevelopmental plasticity changes, and not acute pharmacological effects, would underlie behavioral changes. Neuroplastic effects should be expected to carry over for weeks and may be more reliably detected by searching for differential effects, depending on whether the active compound is administered during the first or the second period, rather than expecting one week of wash-out to truly bring all CNS parameters back to baseline. Our results provide some support to this line of thought; nonetheless, a one-week wash-out period could be applied in future studies.
(F) Our sample size was dictated by the availability of cases affected with a rare disorder, like PMS. It was sufficient to yield nominal significance for several relevant variables but not enough for a superiority trial design, given the small-to-moderate therapeutic effect (i.e., 0.2-0.3). Future studies should benefit from a greater gap in efficacy between MST and a truly inactive placebo, but given these premises, a sample size of n = 50 appears to be the minimum requirement for a cross-over study of MST. Multicenter studies may be necessary if single rare disorders are targeted.
(G) The vast majority of patients recruited for this study were unable to swallow capsules. In these patients, both active compound and active comparator were administered by opening capsules and dissolving their content in a small quantity of juice or soft drink. We allowed this procedure, because we were indeed aware that severe swallowing difficulties are frequent in PMS patients[88] and also because, in our retrospective study[35], we did not observe a loss of efficacy following this protocol. Nonetheless, we cannot entirely exclude that differences in drug administration strategy may have contributed to the interindividual variability in response recorded in the present trial.
In summary, this study provides further promising evidence of the positive effects of CoQ10 in PMS when administered in association with Vitamin E and Polyvitamin B, which also appear to provide independent synergistic contributions. Mild-to-moderate improvement was recorded in 24/31 (77.4%) PMS patients and concerned primarily with the domains of cognition, responsiveness to environmental stimuli and adaptive functioning, motor skills, and stereotypic behaviors. In addition, the low incidence and mildness of side effects encourage further studies of this therapeutic approach, given the severity of PMS and the lack of available treatments. From a different perspective, the small but tangible relief provided to parental quality of life by the addition of CoQ10 to vitamins appears to be an important added value in a severe genetic syndrome with a major impact on the daily living of family members[89] and on parental stress[90]. Defining the most sensitive and reliable outcome measures represents a major hurdle in the experimental psychopharmacology of neurodevelopmental disorders[91]. This trial has been instrumental in defining the outcome measures most sensitive to small-to-moderate clinical change in this severely-affected population, the optimal duration, and many other methodological aspects of the experimental design, setting the stage for confirmatory targeted RCTs for PMS, ASD, and other neurodevelopmental genetic syndromes. These studies will also benefit from the assessment of biochemical parameters of oxidative stress to explore whether and to what extent they predict clinical response.
DECLARATIONS
Acknowledgments
The authors wish to acknowledge all the families and patients who participated in this study, and the Italian Association for Phelan-McDermid Syndrome (AISPHEM) for partially funding this project. The authors also acknowledge Dr. Marilena Briguglio for contributing to the medical visits during her clinical activity.
Authors’ contributions
Conceptualization, methodology, project coordination and administration: Persico AM
Patient randomization and treatment allocation: Turriziani L, Calabrese G, Di Bella T
Medical visits at T0, T1, and T2: Persico AM, Cucinotta F.
Psychological testing at T0, T1, and T2: Boncoddo M, Bellomo F, Turturo G
Response to medical requests from patients and families: Ricciardello A
Data curation: Ricciardello A, Turriziani L, Calabrese G, Tomaiuolo P, Di Bella T, Cucinotta F, Mirabelli S, Persico AM
Statistical analysis: Banchelli F, Cuoghi Costantini R, Asta L, D’Amico R
Writing - original draft preparation: Cucinotta F, Ricciardello A, Turriziani L, Calabrese G, Tomaiuolo P, Di Bella T
Writing - review and editing: Persico AM, Asta L
Read and agreed to the published version of the manuscript: Persico AP, Ricciardello A, Cucinotta F, Turriziani L, Calabrese G, Tomaiuolo P, Di Bella T, Bellomo F, Boncoddo M, Turturo G, Mirabelli S, Asta L, Banchelli F, Cuoghi Costantini R, D'Amico R
Availability of data and materials
All relevant data is contained within the article. The original contributions presented in the study are included in the article; further inquiries can be directed to the corresponding author/s.
Financial support and sponsorship
This work was partly funded by the Italian Association for Phelan-McDermid Syndrome (AISPHEM).
Conflicts of interest
All authors declared that there are no conflicts of interest. The funders had no role in the design of the study, in the collection, analyses, or interpretation of data, in the writing of the manuscript, or in the decision to publish the results.
Ethical approval and consent to participate
The study was conducted in accordance with the ethical standards of the relevant national and institutional committees on human experimentation and with the Helsinki Declaration. The study protocol was approved by the Ethical Committee of the University of Messina (Messina, Italy) (prot. n. 15/18 approved on 06/18/2018). Written informed consent was obtained from all caregivers for their own and their children's participation.
Consent for publication
Not applicable.
Copyright
© The Author(s) 2023.
REFERENCES
2. Phelan K, Rogers RC, Boccuto L. Phelan-McDermid syndrome. In M. P. Adam (Eds.) et. al., GeneReviews®. University of Washington, Seattle; 2005. Available from: https://www.ncbi.nlm.nih.gov/books/NBK1198/[Last accessed on 10 Jul 2023]
3. Phelan K, McDermid HE. The 22q13.3 deletion syndrome (Phelan-McDermid Syndrome). Mol Syndromol 2012;2:186-201.
4. Kolevzon A, Angarita B, Bush L, et al. Phelan-McDermid syndrome: a review of the literature and practice parameters for medical assessment and monitoring. J Neurodev Disord 2014;6:39.
5. National Organization for Rare Disorders. Phelan-cDermid sndrome. Available from: https://rarediseases.org/rare-diseases/phelan-mcdermid-syndrome/#affected-populations [Last accessed on 7 Jul 2023].
6. Betancur C, Buxbaum JD. SHANK3 haploinsufficiency: a "common" but underdiagnosed highly penetrant monogenic cause of autism spectrum disorders. Mol Autism 2013;4:17.
7. Soorya L, Kolevzon A, Zweifach J, et al. Prospective investigation of autism and genotype-phenotype correlations in 22q13 deletion syndrome and SHANK3 deficiency. Mol Autism 2013;4:18.
8. Sarasua SM, Boccuto L, Sharp JL, et al. Clinical and genomic evaluation of 201 patients with Phelan-McDermid syndrome. Hum Genet 2014;133:847-59.
9. Kohlenberg TM, Trelles MP, McLarney B, Betancur C, Thurm A, Kolevzon A. Psychiatric illness and regression in individuals with Phelan-McDermid syndrome. J Neurodev Disord 2020;12:7.
10. Kolevzon A, Delaby E, Berry-Kravis E, Buxbaum JD, Betancur C. Neuropsychiatric decompensation in adolescents and adults with Phelan-McDermid syndrome: a systematic review of the literature. Mol Autism 2019;10:50.
11. De Rubeis S, Siper PM, Durkin A, et al. Delineation of the genetic and clinical spectrum of Phelan-McDermid syndrome caused by SHANK3 point mutations. Mol Autism 2018;9:31.
12. Disciglio V, Lo Rizzo C, Mencarelli MA, et al. Interstitial 22q13 deletions not involving SHANK3 gene: a new contiguous gene syndrome. Am J Med Genet A 2014;164A:1666-76.
13. Ricciardello A, Tomaiuolo P, Persico AM. Genotype-phenotype correlation in Phelan-McDermid syndrome: A comprehensive review of chromosome 22q13 deleted genes. Am J Med Genet A 2021;185:2211-33.
14. Vondráčková A, Veselá K, Kratochvílová H, et al. Large copy number variations in combination with point mutations in the TYMP and SCO2 genes found in two patients with mitochondrial disorders. Eur J Hum Genet 2014;22:431-4.
16. Costales JL, Kolevzon A. Phelan-McDermid sndrome and SHANK3: iplications for teatment. Neurotherapeutics 2015;12:620-30.
17. Verhoeven WMA, Egger JIM, de Leeuw N. A longitudinal perspective on the pharmacotherapy of 24 adult patients with Phelan-McDermid syndrome. Eur J Med Genet 2020;63:103751.
19. Mantle D, Dybring A. Bioavailability of cenzyme Q(10): an overview of the absorption process and subsequent metabolism. Antioxidants 2020;9:386.
20. Weber C, Bysted A, Hłlmer G. The coenzyme Q10 content of the average Danish diet. Int J Vitam Nutr Res 1997;67:123-9.
21. Hargreaves IP. Coenzyme Q10 as a therapy for mitochondrial disease. Int J Biochem Cell Biol 2014;49:105e111.
22. Matthews RT, Yang L, Browne S, Baik M, Beal MF. Coenzyme Q10 administration increases brain mitochondrial concentrations and exerts neuroprotective effects. Proc Natl Acad Sci USA 1998;95:8892-7.
23. Rötig A, Appelkvist EL, Geromel V, et al. Quinone-responsive multiple respiratory-chain dysfunction due to widespread coenzyme Q10 deficiency. Lancet 2000;356:391-5.
24. Beal MF. Mitochondrial dysfunction and oxidative damage in Alzheimer's and Parkinson's diseases and coenzyme Q10 as a potential treatment. J Bioenerg Biomembr 2004;36:381-6.
25. Papucci L, Schiavone N, Witort E, et al. Coenzyme q10 prevents apoptosis by inhibiting mitochondrial depolarization independently of its free radical scavenging property. J Biol Chem 2003;278:28220-8.
26. Kalayci M, Unal MM, Gul S, et al. Effect of coenzyme Q10 on ischemia and neuronal damage in an experimental traumatic brain-injury model in rats. BMC Neuroscience 2011;12:75.
27. Kumari S, Mehta SL, Milledge GZ, Huang X, Li H, Li PA. Ubisol-Q10 prevents glutamate-induced cell death by blocking mitochondrial fragmentation and permeability transition pore opening. Int J Biol Sci 2016; 12:688-700.
28. Degenhardt F, Niklowitz P, Szymczak S, et al. Genome-wide association study of serum coenzyme Q10 levels identifies susceptibility loci linked to neuronal diseases. Hum Mol Genet 2016;25:2881-91.
29. Somayajulu M, McCarthy S, Hung M, Sikorska M, Borowy-Borowski H, Pandey S. Role of mitochondria in neuronal cell death induced by oxidative stress; neuroprotection by Coenzyme Q10. Neurobiol Dis 2005;18:618-27.
30. Moon Y, Lee KH, Park JH, Geum D, Kim K. Mitochondrial membrane depolarization and the selective death of dopaminergic neurons by rotenone: protective effect of coenzyme Q10. J Neurochem 2005;93:1199-208.
31. Hochberg J, Fortes da Silva FB. Latissimus dorsi myocutaneous flap to restore elbow flexion and axillary burn contracture: a report on two pediatric patients. J Pediatr Orthop 1982;2:565-8.
32. Müller T, Büttner T, Gholipour AF, Kuhn W. Coenzyme Q10 supplementation provides mild symptomatic benefit in patients with Parkinson's disease. Neurosci Lett 2003;341:201-4.
33. Shults CW, Beal MF, Fontaine D, Nakano K, Haas RH. Absorption, tolerability, and effects on mitochondrial activity of oral coenzyme Q10 in parkinsonian patients. Neurology 1998;50:793-5.
34. Cooper O, Seo H, Andrabi S, et al. Pharmacological rescue of mitochondrial deficits in iPSC-derived neural cells from patients with familial Parkinson's disease. Sci Transl Med 2012;4:141ra90.
35. Cucinotta F, Ricciardello A, Turriziani L, et al. Efficacy and safety of Q10 ubiquinol with vitamins b and e in neurodevelopmental disorders: a retrospective chart review. Front Psychiatry 2022;13:829516.
36. Psychiatric Association. Diagnostic and statistical manual of mental disorders. Med Health Care Phil 2014;17:241-244.
37. Soorya L, Leon J, Trelles MP, Thurm A. Framework for assessing individuals with rare genetic disorders associated with profound intellectual and multiple disabilities (PIMD): the example of Phelan McDermid Syndrome. Clin Neuropsychol 2018;32:1226-55.
38. Braconnier ML, Siper PM. Neuropsychological assessment in autism spectrum disorder. Curr Psychiatry Rep 2021;23:63.
39. Jacquemont S, Berry-Kravis E, Hagerman R, et al. The challenges of clinical trials in fragile X syndrome. Psychopharmacology 2014;231:1237-50.
40. Sparrow SS, Cicchetti DV, Balla DA. Vineland adaptive behavior scales: second edition (Vineland II), survey interview form/caregiver rating form. Livonia, MN: Pearson Assessments; 2005. Available from: https://www.walmart.com/ip/Vineland-II-Vineland-Adaptive-Behavior-Scales-Survey-Forms-Manual-Interview-Form-Parent-Caregiver-Rating-Form-Pre-Owned-Unknown-Binding-B001P673SK-Do/702415787 [Last accessed on 7 Jul 2023].
41. Vaughan CA. Test Review: E. Schopler, M. E. Van Bourgondien, G. J. Wellman, & S. R. Love Childhood Autism Rating Scale (2nd ed.). Los Angeles, CA: Western Psychological Services, 2010. J Psychoeduc Assess 2011;29:489-93.
42. Guy W. Clinical global impressions ECDEU assessment manual for psychopharmacology. Rockville, MD: National Institute for Mental Health; 1976. Available from: https://ia600306.us.archive.org/35/items/ecdeuassessmentm1933guyw/ecdeuassessmentm1933guyw.pdf [Last accessed on 7 Jul 2023].
43. Guyatt GH, Townsend M, Berman LB, Keller JL. A comparison of likert and visual analogue scales for measuring change in function. J Chronic Dis 1987;40:1129-33.
44. Jaeschke R, Singer J, Guyatt GH. A comparison of seven-point and visual analogue scales. Data from a randomized trial. Control Clin Trials 1990;11:43-51.
45. Shaffer D, Gould MS, Brasic J, et al. A children's global assessment scale (CGAS). Arch Gen Psychiatry 1983;40:1228-31.
46. Constantino JN, Davis SA, Todd RD, et al. Validation of a brief quantitative measure of autistic traits: comparison of the social responsiveness scale with the autism diagnostic interview-revised. J Autism Dev Disord 2003;33:427-33.
47. Lam KS, Aman MG. The repetitive behavior scale-revised: independent validation in individuals with autism spectrum disorders. J Autism Dev Disord 2007;37:855-66.
48. Aman MG, Singh NN, Stewart AW, Field CJ. The aberrant behavior checklist: a behavior rating scale for the assessment of treatment effects. Am J Ment Defic 1985;89:485-91. PMID: 3993694.
49. Tomchek SD, Dunn W. Sensory processing in children with and without autism: a comparative study using the short sensory profile. Am J Occup Ther 2007;61:190-200.
50. Conners CK, Sitarenios G, Parker JD, Epstein JN. The revised Conners' Parent Rating Scale (CPRS-R): factor structure, reliability, and criterion validity. J Abnorm Child Psychol 1998;26:257-68.
51. Achenbach TM, Rescorla LA. Manual for the ASEBA preschool forms and profiles. Burlington, VT: University of Vermont, Research Center for Children, Youth, and Families; 2000. Available from: https://www.researchgate.net/publication/243771256_Manual_for_the_ASEBA_Preschool_forms_profiles [Last accessed on 7 Jul 2023].
52. Achenbach TM, Rescorla LA. Manual for the ASEBA school-age forms and profiles. Burlington, VT: University of Vermont, Research Center for Children, Youth, and Families; 2001. Available from: https://store.aseba.org/MANUAL-FOR-THE-ASEBA-SCHOOL-AGE-FORMS-PROFILES/productinfo/505/ [Last accessed on 7 Jul2023].
53. McConachie H, Mason D, Parr JR, Garland D, Wilson C, Rodgers J. Enhancing the validity of a quality of life measure for autistic people. J Autism Dev Disord 2018;48:1596-611.
54. The WHOQOL Group. Development of the World Health Organization WHOQOL-BREF quality of life assessment. Psychol Med 1998;28:551-8.
55. R Core Team. R: A language and environment for statistical computing. R Foundation for Statistical Computing, Wien, Austria; 2020.
56. Ponson L, Gomot M, Blanc R, et al. 22q13 deletion syndrome: communication disorder or autism? Transl Psychiatry 2018;8:146.
57. Kann O, Kovács R. Mitochondria and neuronal activity. Am J Physiol Cell Physiol 2007;292:C641-57.
59. Carmeli E, Imam B, Bachar A, Merrick J. Inflammation and oxidative stress as biomarkers of premature aging in persons with intellectual disability. Res Dev Disabil 2012;33:369-75.
60. Valenti D, de Bari L, De Filippis B, Henrion-Caude A, Vacca RA. Mitochondrial dysfunction as a central actor in intellectual disability-related diseases: an overview of Down syndrome, autism, Fragile X and Rett syndrome. Neurosci Biobehav Rev 2014;46 Pt 2:202-17.
61. Alvarez-Mora MI, Rodriguez-Revenga L, Madrigal I, Guitart-Mampel M, Garrabou G, Milà M. Impaired mitochondrial function and dynamics in the pathogenesis of FXTAS. Mol Neurobiol 2017;54:6896-902.
62. Liu J, Wang L, Zhan S, Xia Y, Wang L. Coenzyme Q10 for Parkinson's disease. cochrane database of systematic reviews. Chichester: John Wiley & Sons, Ltd 1996; doi: 10.1002/14651858.CD008150.pub2.
63. Persico AM, Cucinotta F, Ricciardello A, Turriziani L. Autisms. Neurodevelopmental disorders. Elsevier; 2020. pp. 35-77.
64. Rossignol DA, Frye RE. Evidence linking oxidative stress, mitochondrial dysfunction, and inflammation in the brain of individuals with autism. Front Physiol 2014; 5:150.
65. Palmieri L, Persico AM. Mitochondrial dysfunction in autism spectrum disorders: cause or effect? Biochim Biophys Acta 2010; 1797:1130-7.
66. Palmieri L, Papaleo V, Porcelli V, et al. Altered calcium homeostasis in autism-spectrum disorders: evidence from biochemical and genetic studies of the mitochondrial aspartate/glutamate carrier AGC1. Mol Psychiatry 2010; 15:38-52.
67. Chauhan A, Audhya T, Chauhan V. Brain region-specific glutathione redox imbalance in autism. Neurochem Res 2012; 37:1681-9.
68. Rose S, Melnyk S, Pavliv O, et al. Evidence of oxidative damage and inflammation associated with low glutathione redox status in the autism brain. Transl Psychiatry 2012; 2:e134.
69. Frustaci A, Neri M, Cesario A, et al. Oxidative stress-related biomarkers in autism: systematic review and meta-analyses. Free Radic Biol Med 2012; 52:2128-41.
70. Tang G, Gutierrez Rios P, Kuo SH, et al. Mitochondrial abnormalities in temporal lobe of autistic brain. Neurobiol Dis 2013; 54:349-61.
71. Gu F, Chauhan V, Chauhan A. Impaired synthesis and antioxidant defense of glutathione in the cerebellum of autistic subjects: alterations in the activities and protein expression of glutathione-related enzymes. Free Radic Biol Med 2013; 65:488-96.
72. El-Ansary A, Bjørklund G, Chirumbolo S, Alnakhli OM. Predictive value of selected biomarkers related to metabolism and oxidative stress in children with autism spectrum disorder. Metab Brain Dis 2017; 32:1209-21.
73. Yenkoyan K, Harutyunyan H, Harutyunyan A. A certain role of SOD/CAT imbalance in pathogenesis of autism spectrum disorders. Free Radic Biol Med 2018; 123:85-95.
74. Adams JB, Baral M, Geis E, et al. The severity of autism is associated with toxic metal body burden and red blood cell glutathione levels. J Toxicol 2009; 2009:532640.
75. Ghezzo A, Visconti P, Abruzzo PM, et al. Oxidative stress and erythrocyte membrane alterations in children with autism: correlation with clinical features. PLoS One 2013;8:e66418.
76. Gorrindo P, Lane CJ, Lee EB, McLaughlin B, Levitt P. Enrichment of elevated plasma F2t-isoprostane levels in individuals with autism who are stratified by presence of gastrointestinal dysfunction. PLoS One 2013;8:e68444.
77. Rose S, Bennuri SC, Murray KF, Buie T, Winter H, Frye RE. Mitochondrial dysfunction in the gastrointestinal mucosa of children with autism: a blinded case-control study. PLoS One 2017;12:e0186377.
78. McElhanon BO, McCracken C, Karpen S, Sharp WG. Gastrointestinal symptoms in autism spectrum disorder: a meta-analysis. Pediatrics 2014;133:872-83.
79. Frye RE, Cox D, Slattery J, et al. Mitochondrial dysfunction may explain symptom variation in phelan-mcdermid syndrome. Sci Rep 2016;6:19544.
81. Adams JB, Holloway C. Pilot study of a moderate dose multivitamin/mineral supplement for children with autistic spectrum disorder. J Altern Complement Med 2004;10:1033-9.
82. Adams JB, Audhya T, McDonough-Means S, et al. Effect of a vitamin/mineral supplement on children and adults with autism. BMC Pediatr 2011;11:111.
83. Adams JB, Audhya T, Geis E, et al. Comprehensive nutritional and dietary intervention for autism spectrum disorder-a randomized, controlled 12-month trial. Nutrients 2018;10:369.
84. Dolske MC, Spollen J, McKay S, Lancashire E, Tolbert L. A preliminary trial of ascorbic acid as supplemental therapy for autism. Prog Neuropsych Biol Psychiatry 1993;17:765-74.
85. Adams JB, George F, Audhya T. Abnormally high plasma levels of vitamin B6 in children with autism not taking supplements compared to controls not taking supplements. J Altern Complement Med 2006;12:59-63.
86. Mousavinejad E, Ghaffari MA, Riahi F, Hajmohammadi M, Tiznobeyk Z, Mousavinejad M. Coenzyme Q(10) supplementation reduces oxidative stress and decreases antioxidant enzyme activity in children with autism spectrum disorders. Psychiatry Res 2018;265:62-9.
87. Eldridge SM, Chan CL, Campbell MJ, et al. PAFS consensus group. CONSORT 2010 statement: extension to randomised pilot and feasibility trials. Pilot Feasibility Stud 2016;2:64.
88. Matuleviciene A, Siauryte K, Kuiper E, Grabrucker AM. European Phelan-McDermid syndrome guideline consortium. Consensus recommendations on chewing, swallowing and gastrointestinal problems in Phelan-McDermid syndrome. Eur J Med Genet 2023;66:104763.
89. Serrada-Tejeda S, Martínez-Piédrola RM, Máximo-Bocanegra N, Sánchez-Herrera-Baeza P, Pérez-de-Heredia-Torres M. Descriptive analysis of adaptive behavior in Phelan-McDermid syndrome and autism spectrum disorder. Front Neurosci 2022;16:893003.
90. Droogmans G, Vergaelen E, Van Buggenhout G, Swillen A. Stressed parents, happy parents. An assessment of parenting stress and family quality of life in families with a child with Phelan-McDermid syndrome. J Appl Res Intellect Disabil 2021;34:1076-88.
Cite This Article
Export citation file: BibTeX | RIS
OAE Style
Persico AM, Ricciardello A, Cucinotta F, Turriziani L, Calabrese G, Tomaiuolo P, Bella TD, Bellomo F, Boncoddo M, Turturo G, Mirabelli S, Asta L, Banchelli F, Costantini RC, D'Amico R. Coenzyme Q10, Vitamin E and Polyvitamin B: an exploratory double-blind randomized cross-over study in Phelan-McDermid Syndrome. Rare Dis Orphan Drugs J 2023;2:13. http://dx.doi.org/10.20517/rdodj.2023.08
AMA Style
Persico AM, Ricciardello A, Cucinotta F, Turriziani L, Calabrese G, Tomaiuolo P, Bella TD, Bellomo F, Boncoddo M, Turturo G, Mirabelli S, Asta L, Banchelli F, Costantini RC, D'Amico R. Coenzyme Q10, Vitamin E and Polyvitamin B: an exploratory double-blind randomized cross-over study in Phelan-McDermid Syndrome. Rare Disease and Orphan Drugs Journal. 2023; 2(3): 13. http://dx.doi.org/10.20517/rdodj.2023.08
Chicago/Turabian Style
Persico, Antonio M., Arianna Ricciardello, Francesca Cucinotta, Laura Turriziani, Giorgia Calabrese, Pasquale Tomaiuolo, Tiziana Di Bella, Fabiana Bellomo, Maria Boncoddo, Giada Turturo, Silvestro Mirabelli, Lisa Asta, Federico Banchelli, Riccardo Cuoghi Costantini, Roberto D'Amico. 2023. "Coenzyme Q10, Vitamin E and Polyvitamin B: an exploratory double-blind randomized cross-over study in Phelan-McDermid Syndrome" Rare Disease and Orphan Drugs Journal. 2, no.3: 13. http://dx.doi.org/10.20517/rdodj.2023.08
ACS Style
Persico, AM.; Ricciardello A.; Cucinotta F.; Turriziani L.; Calabrese G.; Tomaiuolo P.; Bella TD.; Bellomo F.; Boncoddo M.; Turturo G.; Mirabelli S.; Asta L.; Banchelli F.; Costantini RC.; D'Amico R. Coenzyme Q10, Vitamin E and Polyvitamin B: an exploratory double-blind randomized cross-over study in Phelan-McDermid Syndrome. Rare. Dis. Orphan. Drugs. J. 2023, 2, 13. http://dx.doi.org/10.20517/rdodj.2023.08
About This Article
Special Issue
Copyright
Data & Comments
Data
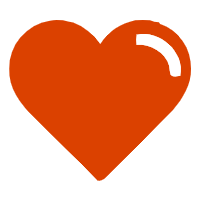

Comments
Comments must be written in English. Spam, offensive content, impersonation, and private information will not be permitted. If any comment is reported and identified as inappropriate content by OAE staff, the comment will be removed without notice. If you have any queries or need any help, please contact us at support@oaepublish.com.